ECG changes in athletes – what is normal and when should I worry?
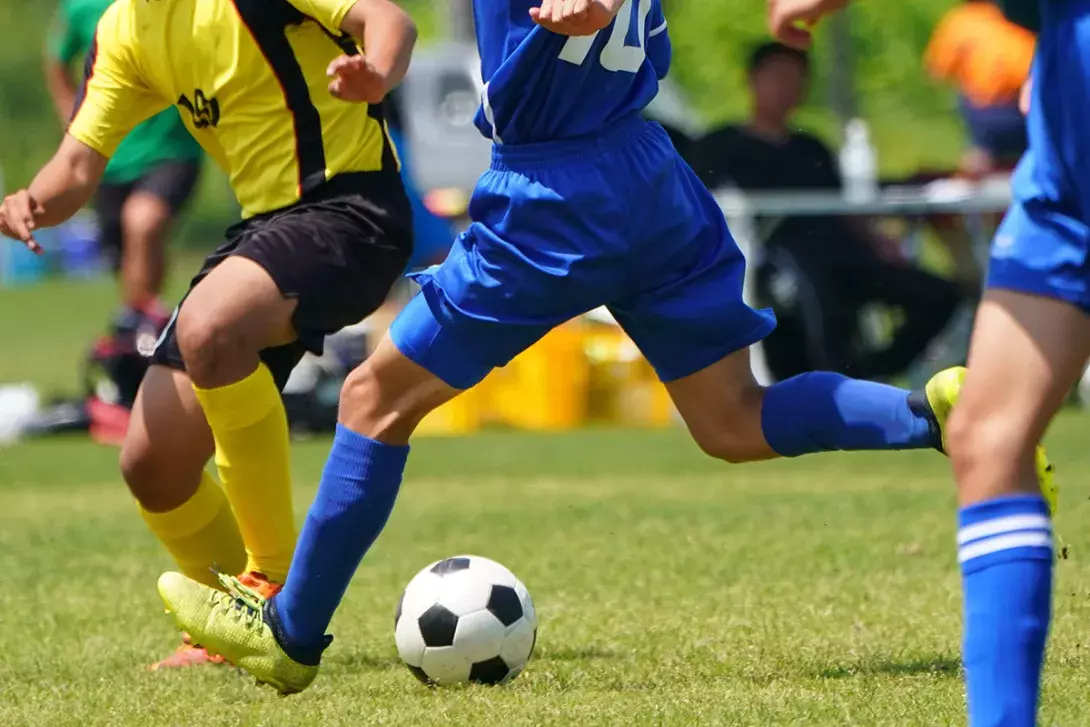
Sudden cardiac death is the leading cause of nontraumatic mortality in athletes. ECG screening is becoming increasingly mainstream. Accurate interpretation of an athlete’s ECG is necessary to ensure that underlying cardiac pathology is detected and managed appropriately, and unnecessary investigations are not pursued.
- Athletes may have an increased risk of sudden cardiac death compared with sedentary individuals of a similar age.
- Underlying structural, genetic and arrhythmogenic cardiac diseases, such as long QT syndrome, hypertrophic cardiomyopathy and arrhythmogenic cardiomyopathy, may predispose an athlete to sudden cardiac death.
- Physiological adaptations to exercise can also occur in the hearts of athletes, which can lead to changes in their ECGs.
- Accurate interpretation of an athlete’s ECG is necessary to ensure underlying cardiac pathology is detected and appropriately managed, and unnecessary investigations are not pursued, which are costly and can cause undue stress for athletes.
- Pre-participation screening, which involves targeted history taking, clinical examination and usually a resting 12-lead ECG, can help identify individuals at risk of underlying cardiac pathology and inform their exercise prescription and ongoing management.
Sudden cardiac death (SCD) is the leading cause of nontraumatic mortality in athletes.1 Paradoxically, athletes may have an increased risk of SCD compared with sedentary individuals of a similar age.2 The prevalence of SCD in the general population has been shown to be 1.3/100,000 among individuals aged 1 to 35 years according to a population-based study in Australia and New Zealand,3 whereas a much higher incidence of SCD of 6.8/100,00 has been shown in adolescent soccer players in the UK.4 Similarly, a study in Italian athletes reported a three times higher incidence of SCD in athletes versus nonathletes.5 A higher rate of sudden death has also been shown in male athletes, black athletes and basketball players.6
Underlying structural, genetic and arrhythmogenic cardiac diseases, such as long QT syndrome (LQTS), hypertrophic cardiomyopathy (HCM) and arrhythmogenic cardiomyopathy (ACM), may predispose an athlete to SCD.7 In addition, physiological adaptations to exercise can occur in the hearts of athletes, which can lead to changes in their ECGs.1 If these ECG changes are misinterpreted, pathology can be missed or unnecessary investigations can be performed, which can cause undue stress for athletes and their families, and are costly for athletes, their clubs and the healthcare system. Therefore, when interpreting an athlete’s ECGs, it is important to differentiate normal physiological changes from underlying cardiac pathology.
Pre-participation screening, which involves targeted history taking, a clinical examination and usually a resting 12-lead ECG, helps identify individuals at risk of underlying cardiac pathology and helps inform their exercise prescription and ongoing management. It is becoming more widely adopted across all sporting codes, with guidelines from the Australasian College of Sport and Exercise Physicians recommending that all athletes participating at an elite level be evaluated for conditions linked to SCD using pre-participation screening.8 Up to 66% of SCD cases in athletes have been shown to be potentially identifiable on a pre-participation screening ECG, accepting that pre-participation screening has never been proven at reducing rates of SCD in athletes.1,9,10 Athletes should be followed up with a trained sports cardiologist if abnormalities are identified on pre-participation screening for further assessment.
ECG changes in athletes
Cardiovascular adaptation to exercise can be seen in athletes who participate in regular physical exercise (minimum of 4 hours per week).1 These adaptations can lead to specific associated changes on the ECG, which may vary depending on gender, race, ethnicity, age, body habitus and intensity or type of sport. Females are the quickest growing demographic in sport and have different cardiovascular adaptations to males. Therefore, a gender-based approach to cardiovascular screening is needed.11
Left ventricular hypertrophy
Left ventricular hypertrophy is considered the most common structural change occurring with intense exercise. Up to a 20% increase in left ventricular wall thickness and cavity size and mass can be seen in some athletes. Typically, this is reflected in the 12-lead ECG as left ventricular hypertrophy meeting voltage criterion (Figure 1 ). This does not warrant further investigation if no other abnormalities are identified on the ECG.1 Some slim athletes may demonstrate an ECG showing higher voltage because of a thinner chest wall and surface electrodes lying closer to the underlying myocardium.
T-wave inversion
T-wave inversion can be normal in leads V1 to V2, III or aVF. Progression in the anterior leads from V1 to V3 can be normal in young adolescent athletes, described as a juvenile pattern, and can also be normal from V1 to V4 in black athletes when found in association with typical upsloping ST elevation (Figure 2 ). However, T-wave inversion in the inferolateral leads (II, III, aVF, V4 to V6, Figure 3 ) represents an abnormal finding. Athletic individuals with abnormal T-wave inversion should undergo echocardiography and be referred to a sports cardiologist for consideration of further testing, such as Holter monitoring and cardiac MRI (Table ).
Abnormal T-wave inversion can be an indication of an underlying diagnosis of HCM or ACM (also known as arrhythmogenic right ventricular cardiomyopathy [ARVC]). HCM should be suspected in athletes with lateral T-wave inversion; however, the T-wave inversion can be widespread, particularly in apical HCM (Figure 4 ). HCM is a genetic condition typically due to pathogenic variants in genes encoding for sarcomeric proteins, leading to increased left ventricular wall thickness, often in an asymmetrical pattern. ACM should be suspected if anterior T-wave inversion is seen beyond V2 in non-black athletes, especially with a flat ST segment. Epsilon wave is a rare finding on an ECG, but can be seen in individuals with ARVC.
ACM is an inherited cardiomyopathy typically due to pathogenic variants in genes encoding for desmosomal proteins, leading to fibrofatty replacement of the myocardium with the individual at risk of malignant arrhythmias triggered from abnormal substrate. It has been shown on recent postmortem studies that 87% of individuals with ACM have some involvement of the left ventricular myocardium; therefore, the disease is no longer considered to be isolated to the right ventricle, and is now more widely accepted to be known as ACM rather than ARVC.12 ACM has been shown to be associated with up to 16 times increased risk of SCD in competitive athletes compared with noncompetitive individuals.12
Endurance and high-intensity exercise has been shown to lead to earlier disease onset and more severe phenotypic expression of ARVC/ACM. This has led to recent guidelines recommending that athletes with ACM abstain from competitive sports.5 This includes athletes who are genotype-positive phenotype-negative (i.e. carry a causative genetic variant but do not yet have any evidence of cardiac phenotype on investigations, such as echocardiography or cardiac MRI).5,13 Both HCM and ACM are genetic conditions, typically inherited in an autosomal dominant manner. Therefore, a diagnosis has implications for first-degree family members, who carry a 50% risk of the same condition.
Sinus bradycardia and atrioventricular block
Sinus bradycardia is common in athletes, especially those who participate in endurance sports, due to increased vagal tone. A resting heart rate as low as 35 beats per minute is accepted in athletic individuals. Atrial ectopic rhythm and wandering pacemaker are normal variants, reflecting an increased vagal tone. First-degree heart block (PR interval >200 ms) does not warrant further investigation, unless the PR interval is severely prolonged (>400 ms). Pauses (especially during sleep) are common in athletes and do not warrant further investigation, unless more than 3 seconds while awake. Second-degree Mobitz type 1 (Wenckebach) block is also considered normal in athletic individuals. Type II second-degree atrioventricular block and complete heart block are not normal, and warrant further investigation.
Prolonged QT interval
The QT interval describes myocardial repolarisation, and should be measured from the start of the QRS complex to the end of the T-wave. The QT interval is best measured in leads II and V5 (excluding U-waves) and is rate dependent, requiring correction using the recommended Bazett’s heart rate correction formula (QTc = QT/square root of R-R interval). Average QT interval ranges differ between sexes, with QTc for females typically measuring longer than those for males. Significant QTc prolongation can be associated with an increased risk of Torsades de pointes and therefore SCD. Significant shortness of the QTc duration of less than 320 ms can be associated with atrial fibrillation or ventricular arrhythmias, and is a very rare genetic condition.
In athletes, a high vagal tone and increased left ventricular mass can prolong the QTc interval. Acceptable upper limits of normal for QTc are less than 470 ms in males and less than 480 ms in females. QTc shortening or prolongation can be caused by use of medications (e.g. antibiotics, antipsychotics, antidepressants) or electrolyte abnormalities, and should prompt a targeted medication history and biochemistry.
A QTc of more than 500 ms is considered diagnostic of LQTS in the absence of acquired causes of QT prolongation (Figure 5 ). LQTS is an inherited arrhythmia syndrome, affecting one in 2000 individuals.1 LQTS is due to pathogenic genetic variants in genes associated with QT prolongation. The three dominant genotypes, KCNQ1 (LQT1), KCNH2 (LQT2), SCN5A (LQT3) represent up to 80% of congenital LQTS diagnoses. The diagnosis should be suspected in an individual athlete with a QTc of more than 470 ms (males) and more than 480 ms (females), especially in the presence of a family history or symptoms, such as unexplained syncope (Box). An exercise stress test is recommended for further investigation, with monitoring of a prolonged recovery period, including measurement of the QTc at 4 minute recovery.1
Brugada ECG patterns
Type 1 Brugada pattern is characterised by a typical coved-shaped ST elevation of more than 2 mm with associated inverted wave (rSr’) in right praecordial leads (Figure 6 ), and is diagnostic of Brugada syndrome if demonstrated on the surface ECG. A type 2 Brugada pattern is typically a saddle back appearance in the right praecordial leads and is not diagnostic of Brugada syndrome. If there is clinical suspicion of Brugada syndrome on the surface ECG, it is recommended to perform an ECG with high lead placement (move the praecordial leads to the 2nd or 3rd intercostal space) to represent the anatomical position of the right ventricular outflow tract. A spontaneous type 1 Brugada ECG pattern requires further investigations irrespective of symptoms and is diagnostic of the Brugada syndrome. Brugada syndrome is an inherited arrhythmia syndrome, with a prevalence of about one in 2000 individuals, which may increase the chance of sudden death (particularly, during sleep). These individuals should be referred to a genetic cardiologist or electrophysiologist for further assessment.
Other ECG patterns
ECG findings that may represent underlying coronary artery disease include pathological Q-waves (Q-wave >25% QRS complex), especially in association with T-wave inversion in same leads, or a left bundle branch block. Coronary artery disease is the leading cause of SCD in older athletes (aged >35 years). A clinical history of exertional chest pain or dyspnoea, especially in the presence of cardiovascular risk factors, warrant further investigation with a cardiologist (Box ). Further investigations include an exercise stress test or exercise stress echocardiography, CT coronary angiography or invasive coronary angiography.
Congenital coronary anatomy anomalies are an important common cause of sports-related SCD. Symptoms such as exertional dyspnoea, syncope, chest pain or arrhythmias are red flags (Box ), which should precipitate further investigations. Baseline ECG may be normal, with further imaging, such as an echocardiography, CT coronary angiography or exercise stress echocardiography, usually required to determine the diagnosis.
Conclusion
Pre-participation screening aims to identify individuals at risk of sports-related cardiac arrhythmias, or even SCD due to underlying cardiac structural or electrical abnormalities, through a combination of thorough clinical history taking, physical examination and resting 12-lead ECG by a specialist trained in sports cardiology. Further evaluation usually by a sports cardiologist is recommended if there are ECG abnormalities or the clinical history or physical examination findings are abnormal. It is important to identify individuals who may be at risk, without overdiagnosing or overinvestigating ECG changes that are normal for an athlete. There are age, sex, sporting type and racial factors that influence an individual’s specific cardiac adaptation to exercise, which need to be considered when assessing the athlete’s phenotype.
If there are questions following pre-participation screening, it is important that all stakeholders (including the athlete, team, club and family) work together with the full sports cardiology multidisciplinary team at an experienced cardio-genetics/sports cardiology centre to ensure appropriate risk-stratification, management and adequate support for the athlete being assessed. CT
COMPETING INTERESTS: None.
References
1. Sharma S, Drezner JA, Baggish A, et al. International recommendations for electrocardiographic interpretation in athletes. J Am Coll Cardiol 2017; 69; 1057-1075.
2. Corrado D, Basso C, Rizzoli G, Schiavon M, Thiene G. Does sports activity enhance the risk of sudden death in adolescents and young adults? J Am Coll Cardiol 2003; 42: 1959-1963.
3. Bagnall RD, Weintraub RG, Ingles J, et al. A prospective study of sudden cardiac death among children and young adults. N Engl J Med 2016; 374: 2441-2452.
4. Malhotra A, Dhutia H, Finocchiaro G, et al. Outcomes of cardiac screening in adolescent soccer players. N Engl J Med 2018; 379: 524-534.
5. Mont L, Pellicia A, Sharma S, et al. Pre-participation cardiovascular evaluation for athletic participants to prevent sudden death: Position paper from the EHRA and the EACPR, branches of the ESC. Endorsed by APHRs, HRS and SOLAECE. Eur J Prev Cardiol 2017; 24: 41-69.
6. Pelliccia A, Sharma S, Gati S, et al. 2020 ESC Guidelines on Sports Cardiology and Exercise in Patients With Cardiovascular Disease: The Task Force on Sports Cardiology and Exercise in Patients With Cardiovascular Disease of the European Society of Cardiology (ESC). Eur Heart J 2021; 42: 17-96.
7. Semsarian C, Gray B, Haugaa K, et al. Athletic activity for patients with hypertrophic cardiomyopathy and other inherited cardiovascular diseases: JACC Focus Seminar ¾. J Am Coll Cardiol 2022; 80:1268-1283.
8. Australasian College of Sport and Exercise Physicians (ACSEP) Position Statement on Pre-participation Cardiac Evaluation in Young Athletes. Reviewed and Endorsed by ACSET Research Committee; 2018.
9. Harmon KG, Asif IM, Maleszewski J, et al. Incidence and etiology of sudden cardiac arrest and death in high school athletes in the United States. Mayo Clinic Proc 2016; 91: 1493-502
10. Harmon KG, Asif IM, Maleszewski J, et al. Incidence, cause and comparative frequency of sudden cardiac death in national collegiate athletic associated athletes: a decade in review. Circulation 2015; 31:10-19
11. Finocchiaro G, Dhutia H, D’Silva A, et al. Effect of sex and sporting discipline on LV adaptation to exercise. JACC Cardiovasc Imaging 2017; 10: 965-972.
12. Miles C, Finocchiaro G, Papadakis M, et al. Sudden death and left ventricular involvement in arrhythmogenic cardiomyopathy. Circulation 2019; 139: 1786-1797.
13. James CA, Bhonsale A, Tichnell C, et al. Exercise increases age-related penetrance and arrhythmic risk in arrhythmogenic right ventricular dysplasia/cardiomyopathy-associated desmosomal mutation carriers. J Am Coll Cardio 2013; 62: 1290-1297.